A Latent Heat Analogue on Ultra-Hot Jupiters
Thermal Dissociation/Recombination of Molecular Hydrogen
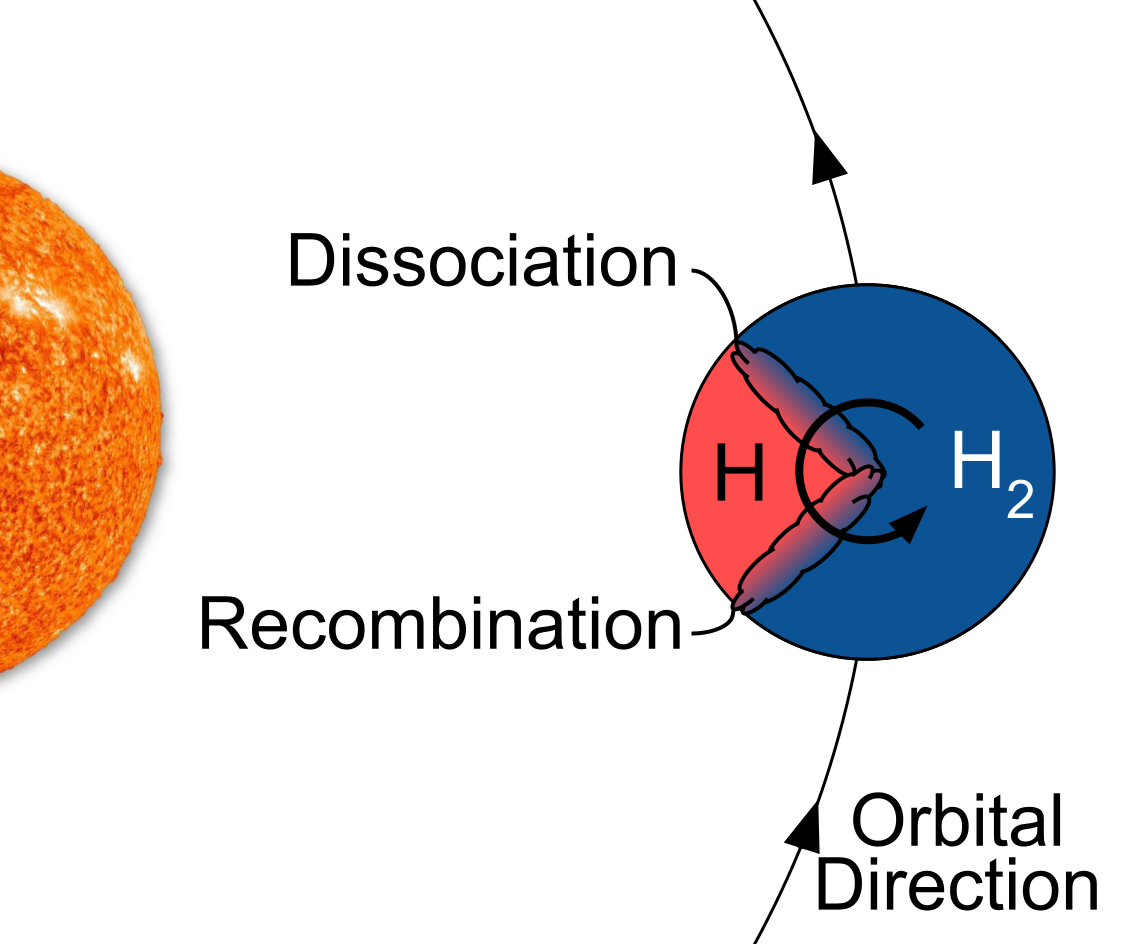
Image Credit: Taylor J. Bell
I am thrilled to share the findings from my recent research on ultra-hot Jupiters, which delves into the dynamic processes governing heat transport in the atmospheres of these scorching exoplanets. Ultra-hot Jupiters are a fascinating subset of exoplanets characterized by their extreme temperatures, which exceed 2030°C (3680°F) on the dayside. These gas giants orbit perilously close to their host stars, resulting in intense stellar irradiation. Traditional models of atmospheric dynamics fall short of explaining the heat distribution observed in Spitzer phase curves of these scorching worlds. Our research aims to bridge this gap by investigating the role of molecular hydrogen (H2) dissociation and recombination on atmospheric heat transport.
Understanding the Impact of H2 Dissociation and Recombination
On ultra-hot Jupiters, the dayside temperatures are so high that the main constituent of the atmospheres, molecular hydrogen (H2), is broken apart ("dissociated") into atomic hydrogen (H). This dissociation absorbs a huge amount of energy, and the inverse process (H2 recombination) is actually used on Earth to perform high-temperature welding. The atomic hydrogen produced by H2 dissociation on the dayside is then transported by winds to the cooler nightside of the planet. As the temperature drops, atomic hydrogen natually recombines into molecular hydrogen, releasing the energy stored during dissociation. This process is fairly similar to the latent heat transport seen in Earth's atmosphere but is much more potent due to the high energy involved in hydrogen dissociation and recombination.
To explore this mechanism, we employed a simple energy balance model incorporating eastward winds. By simulating various scenarios, we demonstrated that the dissociation and recombination of H2 significantly enhances the efficiency of heat transport from the dayside to the nightside of ultra-hot Jupiters. This increased heat recirculation could explain the lower-than-expected temperature contrasts between the dayside and nightside of these planets.
Our findings have significant implications for the modeling of exoplanetary atmospheres. Traditional models that do not account for the effects of H2 dissociation and recombination may overestimate the wind speeds required to transport heat across ultra-hot Jupiters. By incorporating this mechanism, we can achieve a more accurate representation of atmospheric dynamics, leading to better predictions of the thermal structure and weather patterns on ultra-hot Jupiters.
Conclusion
This study is a pivotal step toward a comprehensive understanding of heat transport in extreme exoplanetary environments. However, there is still much to explore. Future research should focus on integrating our findings into more advanced general circulation models to quantitatively assess the impact of H2 dissociation and recombination. Additionally, observations from upcoming missions, such as JWST, will provide valuable data to validate and refine our models.
Our exploration of the role of H2 dissociation and recombination in the atmospheres of ultra-hot Jupiters reveals a complex yet fascinating mechanism of heat transport. This discovery not only enhances our understanding of these distant worlds but also challenges and refines existing atmospheric models. As we continue to uncover the secrets of the universe, studies like this bring us closer to comprehending the diverse and dynamic nature of exoplanetary systems.
Other Links
Link to the Journal ArticleThis blog post was written with the support of ChatGPT-4o